Published in October 2024, by Adam Hiles and the Trisk team.
In a previous post, we discussed how making sterile connections is essential to reliably producing biologics and why the myriad aseptic connectors on the market fall short of enabling the kind of automated interconnection we require to make perfect AAV: namely, they do not adequately prevent microbial ingress outside a well-controlled environment. As a result, developing our own truly sterile connector became a crucial part of the journey to perfect AAV. In this post, we’ll detail the process behind achieving it.
The core challenge
To establish a flow path between sterile volumes, the sterile boundaries of each volume must be breached, typically by removing adjoining sections of their boundaries at some interface, e.g. within a connector. At the critical moment when fluid begins to flow, it is imperative that only the internal sterile surfaces come into contact with the fluid. Traditional methods attempt to achieve this isolation by using deformable elastomeric materials to seal off the non-sterile exterior portions of the removed section as the sterile portions come together to form a new boundary, but we’ve shown in practice that this doesn’t work. In fact, limitations in the thickness and flexibility of available elastomeric materials mean that the sort of failure we observed is inherent in the approach.
With this in mind, we decided that the only way to guarantee sterility was to create a controlled, sterile environment around the connection point itself. This led us to develop a three-step approach:
- Enclose the two mating pairs within a sealed chamber.
- Sterilise the newly formed enclosure.
- Complete the connection within this newly sterilised environment.
Several methods can sterilise a closed chamber, with gamma radiation and steam being the most common. We opted for steam because it is marginally more accessible than the radioactive cobalt-60 required for gamma sterilisation. Steam is already integrated into our facility for the automated turnaround of upstream processing systems.
For the actual part that facilitates the fluidic connection we initially used an off-the-shelf push-to-connect auto valved connector, with future work planned to create our own. Each closed system to be attached is terminated with a mating half and is internally sterilised in prior steps, so that only the external faces need sterilising at this stage of connecting.
Proving the concept
To prove that our approach can create sterile connections, we first built a minimal rig which creates the chamber and allows connection. For this we utilised a spring lost motion mechanism: a single drive first causes the sterilisation chamber to seal around the connectors, and then, upon further actuation, compresses the connectors causing them to mate and allowing fluid to flow. A cross section of the design and images from the actual rig are shown below.
With this rig, we carried out functional microbial challenge testing of the system via the rinse-through and membrane filtration method (similar to USP <71>). We first contaminated the chamber with DH5α (E. coli) bacterial culture. Afterwards, we followed the three step connection method, steam sterilising the chamber at 121°C for 15 minutes. Once connected we rinsed sterile fluid both through the connectors and the sterilised chamber. We captured the rinse flow-through via a 0.45 µm pore size membrane filter and incubated the filter on an agar plate. We repeated the same procedure without the steam sterilisation as positive control. The resulting plates show that contamination is detectable by our challenge method both in the chamber and the product line, and that the steam sterilisation successfully eliminates the contamination
To further challenge the rig’s sterilisation capability, we ran a second test using Geobacillus stearothermophilus (ATCC 7953). These spores are significantly more resistant to heat, making this test a much more stringent measure of sterilisation efficacy. We ran the sterilisation cycle for varying durations of 0, 5, 15 and 30 minutes with the spores contained in an enveloped strip within the chamber. We then incubated in permissive growth media at 60°C for 3 days. As shown below, all results with steam showed complete inactivation of the spores.

Scaling up: the multi-connection challenge
A single sterile connection is useful, but a successful cell culture operation requires many connections. This leads to an idealised solution of multiple connection chambers, with one common steam inlet and outlet to the array and all chambers in parallel to reduce the risk of chamber to chamber cross contamination.
After evaluating a number of concepts, our favoured method to obtain this arrangement was to consolidate all the routing complexity into a single distribution block. Long distribution manifolds were created by gun drilling a grid and then cross drilling to enter and exit each chamber.
The main concern with the manifold flow paths is to ensure they are balanced enough that each chamber reaches sufficient sterilisation temperature. Steam sterilisation achieves these temperatures via saturated steam, wherein both vapour and liquid are present, allowing it to deliver the full latent heat of vaporisation at constant steam temperature. This means that anything cold being heated by the steam will create condensate.
In small diameter pipes, such as in the block, surface tension can prevent condensate from draining due to gravity alone and the flow of gas through the pipes is essential for moving condensate on its way and preventing its build-up. The pressure drop posed by pipe friction or path restrictions will be much greater for a liquid than for vapour. This heightens the chance that condensate build up in a chamber restricts flow so much that the easiest route for steam entering the system is to bypass the wet chamber, creating a feedback loop in which condensate in the wet chamber is not carried away, the flow restriction worsens, the chamber gets even colder and wetter, and thus the chamber is not effectively sterilised.
To make balancing the steam feasible, our design provides flexibility to put the steam into any singular or combination of the gun-drilled channels, and likewise for the steam outlet. This allows us to vary the steam supply and drain configurations to achieve the most consistent heating. To find the optimal steam distribution configuration, we monitored each chamber with a thermocouple. In these tests, we applied lower energy steam to exacerbate the effect of steam imbalance.
In the plots below you can see the effect of injecting into the manifold running along the x=0 chambers (top) and x=2 chambers (bottom).
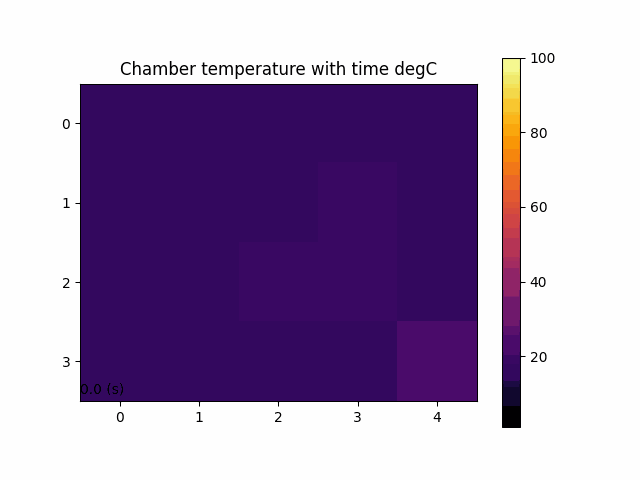
.gif?w=551.9921875)
By trialling these low power steams we found that having the main steam injection points in line with the x=0 channel and x=4 channel provided the most even heat distribution. This makes sense, as heating both outer sides sandwiches the cold central zone, causing it to slowly raise in temperature. In contrast, with a central injection, the outer parts of the block remain cool and sink the thermal energy away, preventing successful heat up. When run at full power, this arrangement achieves the result below, which reaches full sterilisation conditions in less than 7 minutes.
A question of time
One drawback to the singular large block is that, after sterilisation is complete, the thermal dissipation is very slow. For some additions to our process (filling the bioreactor before adding cells, reagents for pH control) the connection time does not matter so we can wait for it to cool before moving fluids. Other reagents have critical time dependence: the time cells spend outside an incubator prior to entering the bioreactor and the complexation time of plasmids and reagents for transfection can have a huge impact on output product quality. The long cooldown time below shows that our block solution is not suitable, and for these cases we need a rig designed with fast cooling in mind.
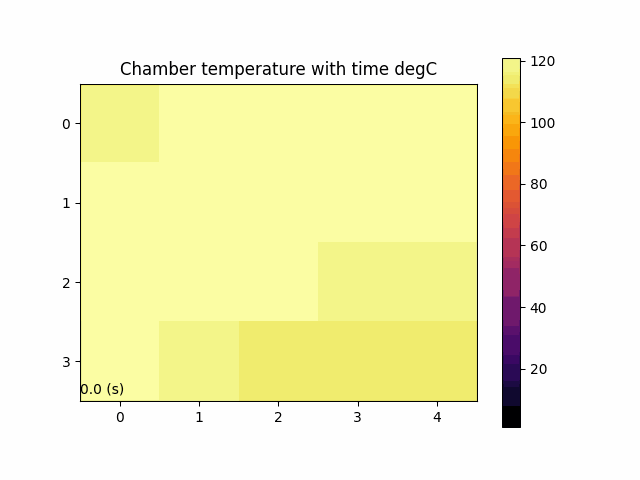
The main problem with cooling a sterile chamber is that any fluid used to cool the chamber increases the risk of contamination. It is therefore ideal to cool a chamber by enclosing the exterior walls with a water jacket. The minimum number of connectors for an addition is two, one to dispense the liquid and one to provide sterile air preventing air locking as the dispense occurs (or provide positive pressure and use this to drive the liquid out). Limiting the quick cool rig to the minimum number of chambers is beneficial to increase the external surface area that can dissipate heat.
The water jacket design presents a challenge because the connection chamber requires four key elements: a steam inlet, a steam outlet, and an axial connector at each end. Since both ends of the chamber are occupied by connectors, and we want to avoid having steam supplies on both connected systems, the steam connections must pass through the water jacket to reach the inner chamber.
Our design achieves this by having the outer wall of the cooling jacket provide a seal to the fittings we use for our steam attachment. Combined with the seal between the fitting and the chamber wall we maintain the integrity of the water jacket. The flow paths relevant to the fast cooling rig are detailed below.
Once the fluid routing was sorted, the rest of the design was relatively benign. The fast cooling rig is rated to higher working temperatures and pressures than the multi-cavity variant, allowing for 3-minute steam sterlisation cycles at 135°C. After sterilisation is complete, water cooling the chamber with room temperature water drops the temperature below 30°C in 7 minutes, making a full cycle time of 11 minutes when accounting for a 1 minute heat up period.
Sterility at scale
With the multi-connector block balanced and the fast cooling rig working, our upstream system is ready to make the connections it needs to produce perfect AAV. The final system can make up to 10 reagent connections and provides in-use steamed connections that enable cell inoculation and plasmid transfections with complexation times as short as 15 minutes — without compromising sterility, even in uncontrolled environments.